A Focus on Low-Energy Atmospheric Neutrinos
Where did we leave off? We have low-energy, atmospheric neutrinos coming from cosmic rays hitting Earth’s atmosphere. On the other hand, we have high-energy astrophysical neutrinos coming from violent events in space.
IceCube is designed primarily to detect high-energy astrophysical neutrinos. But other scientists (such as Michael Larson here at the Pole) are interested in exploring low-energy atmospheric neutrinos.
Flavors of Neutrinos
There are three “flavors” of neutrinos – an electron neutrino, a muon neutrino, and a tau neutrino. You can think of these as flavors of ice cream – strawberry, chocolate, and vanilla are all flavors of ice cream, they just vary a bit in taste.
We didn’t always know about these three flavors though. In the 1960s, we only knew about electron neutrinos. A famous experiment, called Homestake, was designed to measure these neutrinos.
A giant tank full of essentially cleaning fluid was built deep in the Homestake mine in South Dakota. Because it is in a mine, non-neutrino particles could be filtered out (just like how IceCube uses the Earth as a filter for particles coming from the North Pole). If a neutrino were to strike an atom in the cleaning fluid, that atom would change from Chlorine to Argon. A filtration system would capture that Argon atom. This really was an incredible experiment – from this massive tank of fluid, about six atoms needed to be extracted in order to detect a neutrino.
What the Homestake experiment found was surprising. In comparison to the theoretical predictions (based on the fusion in the center of the sun), only about 1/3 of the expected neutrinos were being detected.
For nearly two decades scientists figured there must be something wrong with this experiment. But over and over again, they’d get 1/3 of the expected neutrinos. Other experiments were also built, and the results were reproducible. The experimental setup wasn’t the problem. What gives?
If it wasn’t an issue with the experiment, it must have been an issue with the theory. Did we not fully understand fusion in the sun? Or did we not fully understand neutrinos? Scientists argued back and forth until a man named John Bahcall convincingly said (more or less), “No! The fusion models are correct! We must not understand neutrinos.”
Scientists returned to an old idea – what if there were different flavors of neutrinos? What if neutrinos could oscillate back and forth between these flavors?
In the early 1990s, experiments started to measure muon neutrinos and this flavor idea had some evidence to back it up. Later in the 1990s, tau neutrinos were measured as well. And thus we have the three flavors of neutrinos – electron, muon, and tau neutrinos – that we know and love.
Scientists now understand why the Homestake experiment only saw 1/3 of the expected neutrinos – it was only sensitive to one of the three flavors of neutrinos (electron neutrinos).
In 2001, an experiment sensitive to all three flavors was built in Kamioka, Japan. The scientists on this Kamiokande experiment won the Nobel Prize for their achievement of definitively showing that neutrino oscillations were necessary.
Since, Super-Kamiokande (a larger version of Kamiokande) has been built, and Hyper-Kamiokande (an even larger version) is in the works.
Neutrino Oscillations
We now believe that neutrinos oscillate between flavors. For example, let’s say a cosmic ray hits the atmosphere at the North Pole and produces a muon neutrino. That neutrino will travel all the way through the Earth until it reaches the South Pole. But it does not pop out the same as it went in. Instead, this formerly muon neutrino is now a tau neutrino.
Why do these neutrino oscillations occur? When do they occur? How do they occur? These are the driving questions behind DeepCore.
DeepCore
In the center of IceCube sits an additional 8 strings holding 60 DOMs each, creating a higher density of DOMs than elsewhere in IceCube. We call this region DeepCore.
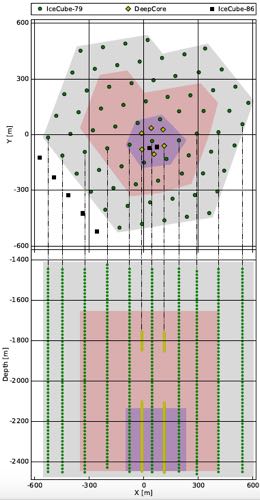
While IceCube is designed for high-energy astrophysical neutrinos, DeepCore is designed for low-energy atmospheric neutrinos.
Atmospheric neutrinos have much lower energies and are therefore only capable of producing dim light. DeepCore is built in the clearest ice and also has the highest density of DOMs, making it ideal to detect this dim light.
Fun fact: DeepCore is indeed this densely DOM’d area, with the exception of “the dust layer.” Here there’s a volcanic dust layer that formed tens of thousands of years ago. It’s about 100m thick! No DOMs were deployed here because the dust would obscure light.
Detecting Flavors of Neutrinos
Collaborating with theorists around the world, we know the flavor of a neutrino when it’s created in the North Pole sky. Using DeepCore, we can tell what flavor the neutrino pops out as here at the South Pole.
Each flavor of neutrino has a specific pattern when it lights up the DOMs.
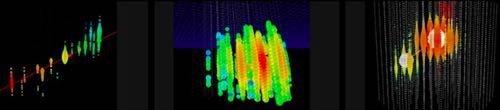
- Muon neutrinos create a cascade followed by a beam (called a track)
- Electron neutrinos create a ball of light (called a cascade)
- Tau neutrinos create two cascades
In the simulation below, you will first see a muon neutrino (as a track), then you’ll see an electron neutrino being detected (as a cascade). These are real detections!
Results (so far)
The first round of analysis of data coming from DeepCore has been analyzed, and the results proved consistent with similar experiments around the world. Next up is a round of improvements to DeepCore. One specific area of focus in the future will be looking for tau neutrinos, which haven’t been detected before! If we can detect a tau neutrino, we have another new way of looking at our universe.
Add new comment